Plasma membrane expression of WT and mutant PTH1Rs
The positions in the PTH1R of the three studied mutations of Eiken syndrome, E35K, Y134S and R485X, as well as the H223R mutation of JMC are shown in Fig. 1a and b. Cell surface expression of the receptors in transiently transfected HEK293-derived Gs22a cells was assessed via antibody binding to an HA epitope tag incorporated into the non-essential E2 region of the receptor’s ECD27. The PTH1R-E35K mutant was expressed at levels comparable to those of PTH1R-WT, while the other mutants were expressed at 30-70% of the level of PTH1R-WT (Fig. 1c and Supplemental Fig. 1). These expression assays and subsequent functional assays were performed using an equivalent amount of plasmid DNA for each PTH1R variant (100 ng/well in 96-well plate assays), which provided the maximum level of expression possible for each variant, as indicated by the results of DNA-titration experiments (Supplemental Fig. 2a, b).
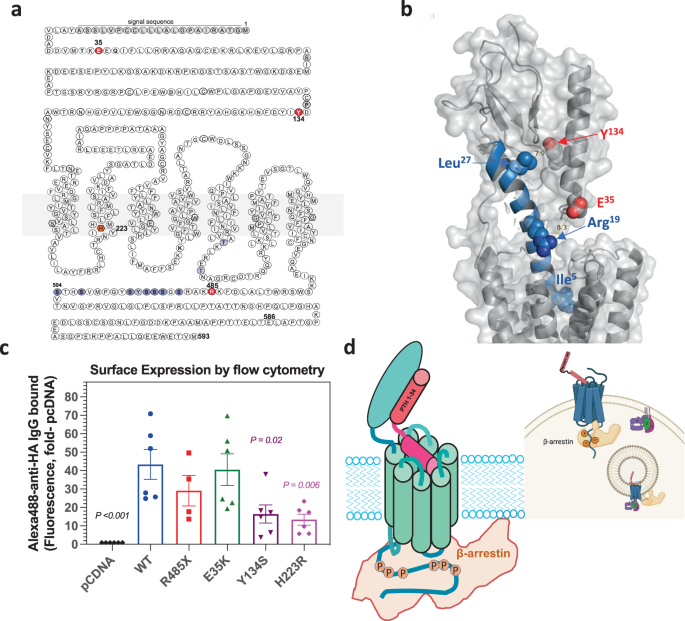
a Map of the PTH1R with sites of three studied Eiken mutations (E35K, Y134S and R485X) shaded red; the site of the Jansen disease mutation (H223R) shaded orange, and sites of C-tail serine phosphorylation shaded blue. b 3-D view of the PTH1R in complex with LA-PTH {cryo-EM structural file PDB.6nBF, ref. 69} showing the extracellular domain (ECD) and upper portion of the transmembrane domain (TMD) regions of the complex with receptor shaded gray and ligand shaded blue. Sidechain atoms of E35 and Y134 in the PTH1R ECD, and Leu27, Arg19, and Ile5 in the ligand are displayed as spheres with oxygens colored red and nitrogens colored blue. Yellow dashed lines indicate measured distances between side chain oxygen of E35 and the proximal sidechain nitrogen of Arg19 (8.3 Å) and between the side chain oxygen of Y134 and the proximal sidechain carbon of Leu27 (7.9 A˚). c Surface expression levels of the HA-tagged PTH1R variants in transiently transfected Gs22a (HEK293/glosensor) cells measured by immunofluorescence flow cytometry. Mean total cell fluorescence levels are normalized to values in cells transfected with pCDNA3.1. Bar heights indicate means ± SEM of four to six experiments and data points indicate measurements from each separate experiment. P values indicate Student’s t test comparisons to PTH1R-WT. Gating strategies are as described in Supplemental Fig. 11. d Schematic of a possible mode of PTH1R complexing with PTH(1-34) or PTHrP(1-36) ligand and β-arrestin, which utilizes two key predicted sites of receptor contact involving (1) the N-terminal portion of β-arrestin and phosphorylated (P) serine or threonine residues in the PTH1R C-tail, and (2) the finger loop domain near the center of β-arrestin and the TMD core of the receptor. The inset depicts possible modes of interaction of the receptor with arrestin at the plasma membrane and in endosomes, as suggested by studies on other GPCRs31,70. The inset graphic was generated using BioRender.com.
Basal cAMP generation and effects of β-arrestin2 overexpression
Basal levels of cAMP generation monitored via the glosensor reporter stably expressed in Gs22a cells28 were markedly elevated in cells expressing PTH1R-R485X relative to those in cells expressing PTH1R-WT and were comparable to those observed for PTH1R-H223R (Fig. 2a and Supplemental Fig. 2b). Modest yet consistent elevations in basal cAMP were observed in cells expressing PTH1R-E35K, while basal cAMP levels for PTH1R-Y134S were comparable to those for PTH1R-WT. The basal cAMP signaling activity of PTH1R-R485X was similar to that observed for PTH1R-PD, in which serine residues at positions 489, 491, 492, 493, 495, 501 and 504 in the C-tail are replaced by alanine11,28, whereas the basal signaling activity of PTH1R-546X, in which the C-tail is truncated at a site downstream of the phosphorylation cluster was comparable to that of PTH1R-WT (Fig. 2b). These results confirm prior findings of elevated basal cAMP signaling for PTH1R mutants lacking serine-threonine phosphorylation residues in the mid-region of the receptor’s C-tail14,28 and also establish that the increase in basal signaling of PTH1R-R485X is due to the absence of these residues and not to the loss of other potential docking determinants in the more distal C-tail region, as used, for example, by NHERF scaffolding proteins29,30.
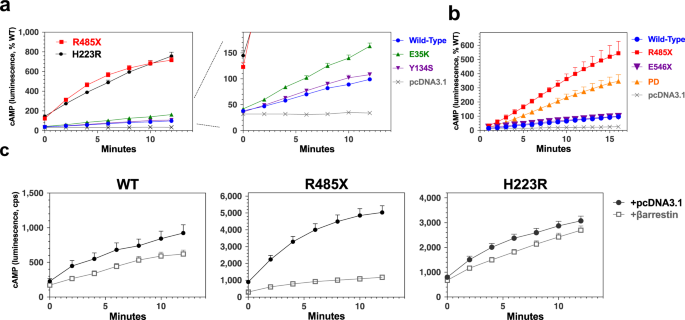
a Basal intracellular cAMP generation assessed in Gs22a (HEK293/glosensor) cells transiently transfected with plasmids to express PTH1R-WT, PTH1R-R485X, PTH1R-E35K, PTH1R-Y134S, or PTH1R-H223R, or with pCDNA3.1; shown are changes in glosensor-derived luminescence over time after addition of luciferin (t = 0). Signals are normalized to the peak signal observed with PTH1R-WT (100%). Peak signals attained for PTH1R-R485X, PTH1R-E35K, PTH1R-Y134S, and PTH1R-H223R, as per-cents of the PTH1R-WT peak, were 719 ± 72 (P ≤ 0.0001), 163 ± 16 (P = 0.0023), 111 ± 12 (P = 0.39) and 756 ± 125 (P ≤ 0.00036), respectively. b Basal cAMP signaling in Gs22A cells expressing PTH1R–PD (phosphorylation deficient), in which serines at position 489, 491, 492, 493, 494, 496, and 504 in the C-tail are replaced by alanine, and PTHR-E546X, which is truncated at position 546, normalized to PTH1R-WT (100%). c Basal cAMP signaling in Gs22A cells co-transfected with plasmids encoding a PTH1R (WT or mutant) and either pCDNA3.1 or β-arrestin2YFP; luminescence signals as counts per second (cps) are plotted vs. time after addition of luciferin (t = 0). The area-under-the-curve (AUC) of the responses for co-transfection with pCDNA3.1 vs. with β-arrestin2YFP, normalized to the response for PTH1R-WT/pCDNA3.1, for PTH1R-1WT were 100 ± 0 vs. 84 ± 23, P = 0.5; for PTH1R-R485X were 602 ± 36 vs.150 ± 19, P = 0.0004, and for PTH1R-H223R were 466 ± 208 vs. 381 ± 170, P = 0.8 (P values determined by Student’s t test). Data are means (±SEM) of six (a) or three independent experiments with three or more wells in each.
Quite strikingly, co-transfection with β-arrestin2YFP strongly suppressed the basal cAMP signaling activity of PTH1R-R485X, such that the resulting cAMP levels were comparable to those in cells expressing PTH1R-WT (Fig. 2c). These findings imply that that PTH1R-R485X can interact with β-arrestin despite the absence of most of the receptor’s C-tail. This interpretation is consistent with recent cryogenic electron microscopy (cryo-EM) structures of other GPCRs in complex with β-arrestin showing that interactions occur to the TMD core region in addition to the receptor C-tail31,32,33, as well as a recent cross-linking study that identifies specific proximity points between β-arrestin2 and the both the TMD and C-tail regions of the PTH1R26, as depicted in Fig. 1d. Co-transfection with β-arrestin2YFP resulted in more modest, albeit measurable decreases in the basal cAMP signaling activity of PTH1R-WT and PTH1R-H223R. A previous study also found that the constitutive cAMP signaling of PTH1R-H223R could be at least partly suppressed by β-arrestin2 co-transfection12. The marked difference we observe in the capacity of β-arrestin2 to suppress basal cAMP signaling by PTH1R-R485X as compared to that of PTH1R-H223R highlights the distinct mechanisms by which these two mutations lead to increased receptor activity, as the C-tail truncation most likely alters the basal-state interaction with β-arrestins while the H223R mutation in TM2 likely perturbs a conserved polar network that controls receptor activation and deactivation processes23.
Agonist-induced signaling responses of PTH1R variants
We then assessed the capacities of the PTH1R mutants to activate cAMP signaling in response to stimulation by either PTH(1-34) or PTHrP(1-36) (Supplemental Table 1). PTH1R-E35K and PTH1R-Y134S responded to each ligand with potencies and efficacies that were comparable to those observed on PTH1R-WT while PTH1R-R485X exhibited a potency for each ligand that was enhanced 2- to 4-fold relative to that on PTH1R-WT, albeit the difference was significant only for PTHrP(1-36) (Fig. 3a, Supplemental Table 3, and Table 1). The response maximum (Emax) attained by each ligand on PTH1R-R485X was reduced to about 80% of that attained on PTH1R-WT, although the difference was again significant only for PTHrP(1-36). PTH1R-H223R exhibited moderately reduced potencies and efficacies for each ligand, which is consistent with prior findings for this PTH1R mutant34.
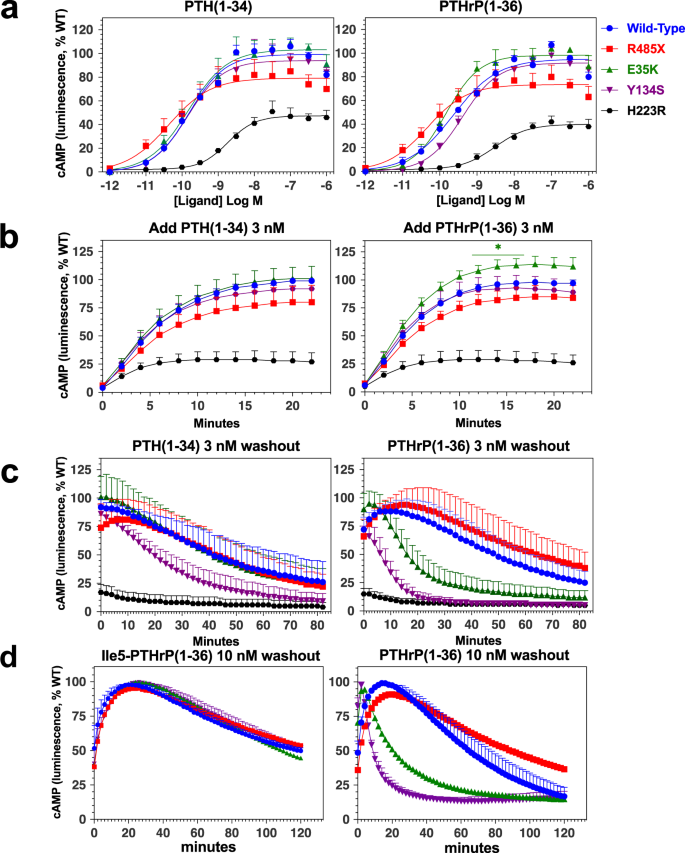
cAMP Signaling responses to PTH and PTHrP ligands were assessed in Gs22a (HEK293/glosensor) cells transiently transfected with PTH1R-WT or a PTH1R mutant. a Dose-responses for PTH(1-34) and PTHrP(1-36). For each ligand concentration on each receptor, the peak luminescence signal, which typically occurred ~15 min after ligand addition, was normalized to the maximum peak signal observed for that ligand on PTH1R-WT (100%) and plotted vs. ligand concentration. b Time courses of cAMP signaling following addition of PTH(1-34) or PTHrP(1-36) at concentrations of 3 nM. The luminescence signals are normalized to the peak signal observed for each ligand on PTH1R-WT (100%); the signals observed for PTHrP(1-36) on PTH1R-E35K at 12–16 min were higher than those on PTH1R-WT (*p < 0.05). c Time courses of cAMP signaling following washout to remove unbound ligand of the cells treated in b. The cAMP-dependent luminescence signals observed for each ligand after washout are normalized to the peak signal observed after washout on PTH1R-WT (100%). d Cells were pretreated for 30 min with Ile5-PTHrP(1-36) or PTHrP(1-36), each at a concentration of 10 nM, and then after washout, cAMP-dependent luminescence signals were recorded and normalized to the peak signal observed for each ligand after washout on PTH1R-WT (100%). Data are means (±SEM) of five (a), four (b, c, e) or three (d) separate experiments.
Comparison of the time-course data for the increases in cAMP generation that occurred in the presence of PTH(1-34) and PTHrP(1-36) at a near-saturating concentration (3.0 nM) revealed that the peak signals attained by PTHrP(1-36) on PTH1R-E35K were moderately but consistently higher than those on PTH1R-WT, whereas the peak signals attained by PTH(1-34) on this mutant receptor were comparable to those on PTH1R-WT, as were those for each ligand on the other mutant receptors (Fig. 3b). After washing away the unbound ligand, the cAMP signals induced by PTH(1-34) decayed at similar rates in cells expressing PTH1R-WT, PTH1R-E35K or PTH1R-R484X, while those in cells expressing PTH1R-Y134S decayed at a moderately faster rate, as compared to with PTH1R-WT. For the cAMP signals induced by PTHrP(1-36), the rates of decay were noticeably faster for both PTH1R-E35K and PTH1R-Y134S, as compared to those for PTH1R-WT or PTH1R-R485X. Thus we observed marked reductions in the duration of the signaling responses induced by PTHrP(1-36) on both the PTH1R-E35K and PTH1R-Y134S variants, which suggests that despite a rapid initial onset of the signaling response, the active ligand-receptor formed by this ligand and these two receptor variants are relatively unstable.
Divergent residues at positions 5 and 19 in PTH and PTHrP as determinants of altered ligand interactions on PTH1R Eiken mutants
The cAMP time course data of Fig. 3b and c suggest that both the E35K and Y134S PTH1R mutations exert distinct effects on the interaction with PTH(1-34) versus PTHrP(1-36). Thus, while PTHrP initially activates each mutant receptor at least as efficiently as it does PTH1R-WT, the mutant complexes formed with PTHrP are less stable as compared to those formed with PTH, and also to those formed by PTHrP on PTH1R-WT. One notable site of amino acid divergence in the two ligands is position-5, which is Ile in PTH and His in PTHrP. These residues are known to be key determinants of altered selectivity effects that have been observed for these ligands on PTH1R-WT, as the His5–>Ile substitution in PTHrP peptides markedly enhances receptor binding affinity, likely due to a more optimal interaction of the Ile side chain with hydrophobic residues in the orthosteric pocket of the receptor’s TMD region3,4,5,35. Consistent with this, we observed that Ile5-PTHrP(1-36), as compared to His5-PTHrP(1-36) induced a more sustained cAMP signaling response on each PTH1R variant, including PTH1R-E35K and PTH1R-Y134S (Fig. 3d).
We also investigated ligand residue 19, which diverges as positively charged arginine in PTHrP and negatively charged glutamate in PTH, and is known to be a second important determinant of ligand selectivity at the PTH1R36. The cryo-EM structure of the LA-PTH/PTH1R complex shows that Arg19 in LA-PTH is relatively close (~8 A˚ at closest side chain atoms) to Glu35 in the receptor (Fig. 1b); a proximity also observed in the more recent cryo-EM structures of the PTH1R in complex with native PTH(1-34) and PTHrP(1-34) peptides4. As Glu35 is replaced by positively charged lysine in PTH1R-E35K, we assessed whether the differences in cAMP signaling actions we observed for PTH and PTHrP peptides on this variant might involve charge-based differences in interactions between the sidechains of the residue at position 19 in the ligands and at position 35 in the receptor. We used for these studies two pairs of PTH probe peptides, PTH(1-31) and Mc-PTH(1-34) for which in each pair position 19 was either Glu or Arg (Supplemental Table 1). Stimulation of cells expressing PTH1R-E35K revealed for each peptide pair a small, yet consistent enhancement in the cAMP signaling response induced by the peptide containing arginine at position 19, relative to that containing Glu-19 (Supplemental Fig. 3). Although these differences did not attain statistical significance, they nevertheless support a model by which the E35K mutation confers different effects on the interaction with PTH vs PTHrP due to altered interaction with the divergent residue-19 in the ligand. The mild enhancing effect on initial signaling observed for PTHrP on PTH1R-E35K, as well as the increase in the rate of decay of the response, would thus arise from a charge-based repulsive interaction between the positively charged sidechains of Arg-19 in the ligand and that of Lys-35 in the mutant receptor that facilitates initial activation but leads to a relatively unstable complex. For PTH, the negatively charged sidechain of Glu-19 provides a more complementary charge-based interaction with the Lys-35 sidechain that does lead to such changes in the onset of activation and the rate of decay of the signaling response. However, the precise mechanisms underlying such dynamic processes of ligand induced signaling and deactivation at the PTH1R remain to be elucidated.
Preserved iCa signaling in PTH1R-R485X
It was previously suggested that the delayed ossification seen in Eiken syndrome patients with the PTH1R-R485X variant might arise from an impaired capacity of the mutant receptor to activate the Gαq/phospholipase C (PLC)/inositol triphosphate (IP3)/intracellular calcium (iCa2+) signaling7. This hypothesis was based on the moderately delayed bone mineralization observed in mice expressing a PTH1R mutant altered in intracellular loop 2 (E317KKY320– > DSEL) that is specifically impaired for Gαq/PLC/iCa2+/IP3 signaling37. We therefore examined iCa2+ signaling for the PTH1R Eiken variants and found that PTH1R-R485X as well as PTH1R-E35K, produced responses to either PTH(1-34) or PTHrP(1-36) that were at least as robust as those produced by PTH1R-WT (Supplemental Fig. 4). The results therefore do not support an impairment in iCa++ signaling as a major determinant of the Eiken syndrome phenotype in patients with either of these two mutations. We observed only a weak if any increase in iCa2+ signaling for PTH1R-Y134S and PTH1R-H223R. A defect in PLC/IP3/iCa2+ signaling for PTH1R-H223R is consistent with prior findings on this mutant PTH1R that causes JMC38. The defect in iCa2+ signaling observed for PTH1R-Y134S could be due in part to a reduced level of surface expression (Fig. 1c and Supplemental Fig. 1c)39. Another potential factor, however, is that on the PTH1R-Y134S mutant there is a faster rate of ligand dissociation, as compared to on PTH1R-WT, which is suggested by the faster rate of decay of the cAMP response observed for the Y134S mutant after ligand washout (Fig. 3c and Supplemental Table 3). It therefore remains possible that alterations in both cAMP and IP3/iCa2+ signaling responses to PTH and/or PTHrP ligands contribute to the clinical phenotype in patients with the PTH1R-Y134S variant.
Radioligand binding properties of PTH1R mutants
We assessed the capacities of the PTH1R variants in intact GS22a cells to bind 125I-PTHrP(1-36) as well as the higher affinity control radioligand 125I-LA-PTH*40. The total binding of 125I-PTHrP(1-36) on PTH1R-R485X and PTH1R-H223R was comparable to that on PTH1R-WT but was reduced by ~50% of PTH1R-WT on PTH1R-E35K and PTH1R-Y134S (Fig. 4a). The reductions in binding of 125I-PTHrP(1-36) to PTH1R-E35K and PTH1R-Y134S were specific for that radioligand, as total binding of 125I-LA-PTH* was similar on each PTH1R variant (Fig. 4b). Competition binding assays performed using 125I-LA-PTH* as tracer radioligand and unlabeled PTH(1-34) competitor ligand revealed similar apparent affinities for PTH(1-34) on each PTH1R Eiken variant (Fig. 4c). The apparent affinity of PTH(1-34) on PTH1R-H223R was enhanced ~13-fold versus that on PTH1R-WT, which is consistent with prior studies showing enhanced affinities for PTH agonist ligands on the constitutively active PTH1R-H223R mutant41. Unlabeled PTHrP(1-36) exhibited little if any capacity to inhibit binding of 125I-LA-PTH* to even PTH1R-WT, and so apparent affinities for this peptide were not determined. Overall, these binding data are consistent with the notion that the E35K and Y134S PTH1R mutations selectively reduce overall affinity for PTHrP, which in turn leads to relatively more rapid rates of decay in the cAMP responses after initial complex formation (Fig. 3c, d).
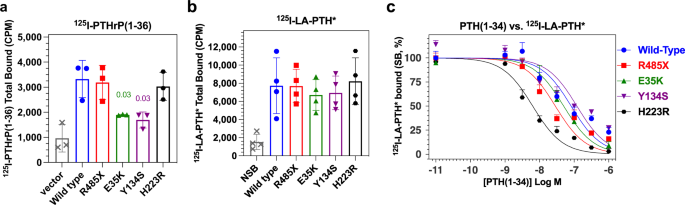
Binding was assessed in intact Gs22a cells transiently transfected with plasmids encoding a PTH1R variant or with pCDNA3.1 (vector). a Total binding of 125I-PTHrP(1-36). Bar heights indicate the means ± SEM of three individual experiments represented by the data points. P values are shown for significant differences vs. PTH1R-WT. b Total binding of 125I-LA-PTH*; wells containing a saturating concentration of PTH(1-34) were used to determine non-specific binding (NSB). c Competition binding of unlabeled PTH(1-34) versus the 125I-LA-PTH* tracer radioligand. The total specific binding (SB) at each PTH(1-34) concentration is normalized to the maximum SB observed at each receptor (100%) and plotted versus PTH(1-34) concentration. Curves were fit to the data using a non-linear regression equation; resulting pIC50 and relative maximum binding parameters are reported in Supplemental Table 4. Data are means (±SEM) of three (a) or four experiments.
Impaired agonist-induced β-arrestin2 recruitment by PTH1R Eiken mutants
We then evaluated the effects of the mutations on the capacity of the PTH1R to recruit β-arrestin2 and internalize with it to endosomes in response to agonist-stimulation. For this purpose, we transiently transfected the PTH1R variants into HEK293-derived GBR24 cells stably expressing β-arrestin2YFP 40,42 and then treated the cells with PTH(1-34)TMR for 30 min before fixing and visualizing the cells by fluorescent microscopy. We observed that for each PTH1R variant PTH(1-34)TMR staining (red) was localized into clusters, which we interpret as ligand-receptor complexes internalized into endosomes, and the clusters formed with PTH1R-WT, PTH1R-E35K, PTH1R-Y134S and PTH1R-H223R were co-localized with β-arrestin2YFP (Fig. 5a, b and Supplemental Fig. 5a). In contrast, β-arrestinYFP did not co-localize in clusters with PTH(1-34)TMR in cells expressing PTH1R-R485X, but instead remained dispersed in the cytoplasm.
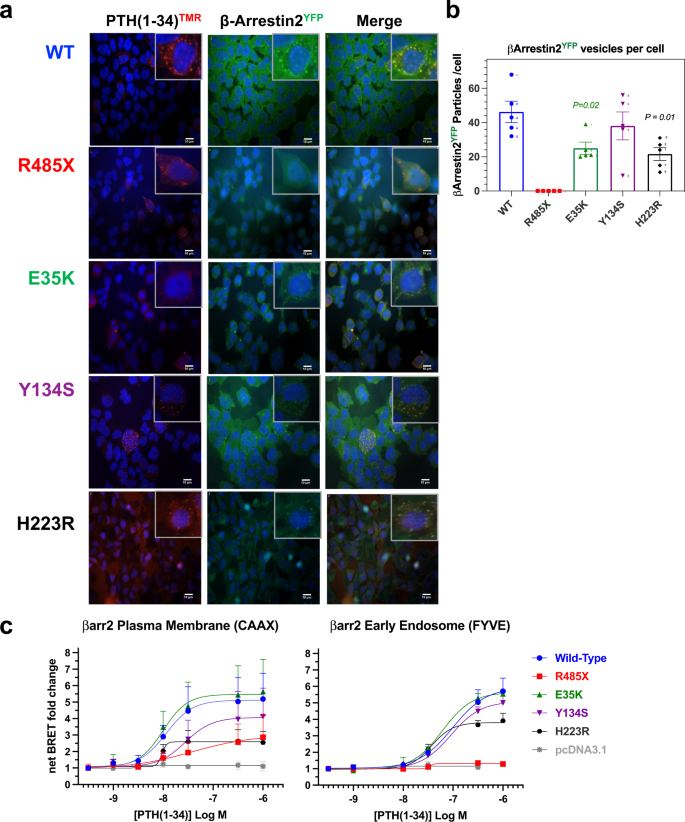
a GBR-24 (HEK293/β–arrestin2YFP stable) cells42 transiently transfected to express the PTH1R-WT or a PTH1R mutant were treated with PTH(1-34)TMR (10 nM) for 30 min, then rinsed, fixed and imaged by fluorescence microscopy. The insets show 3X-expanded views of selected cell regions to highlight the co-localization of PTH(1-34)TMR (red) with β–arrestin2YFP (green) into distinct clusters, interpreted as endosomes, in cells transfected with PTH1R-WT, PTH1R-E35K, PTH1R-Y134S, and PTH1R-H223R, and the absence of such co-localization with β–arrestin2YFP into clusters with PTH1R-R485X. Cells that exhibit only diffuse green fluorescence for β-arrestin2yfp and/or no TMR-PTH ligand red fluorescence are interpreted as cells not transfected with a PTH1R plasmid, which serve as internal negative controls42,71. Images are representative of three independent experiments. Scale bars indicate 10 μm. b ImageJ quantification of β–arrestin2YFP clusters present in the fluorescent microscopy images; bar heights indicate the means ± SEM of counts from five cells shown by the individual data points; P values determined by Student’s t test are shown for significant differences vs. PTH1R-WT. c BRET analyses of β–arrestin2 recruitment in HEK293 cells transiently co-transfected with plasmids encoding a PTH1R variant, β-arrestin2-rLucII as BRET donor, and either the rGFP-CAAX (plasma-membrane) or rGFP-FYVE (early endosome) as BRET acceptor. BRET signals were measured upon addition of ligand with luciferin analog (prolume purple) and the maximum signal observed ~20 min after addition for each PTH1R variant was normalized to the signal observed in the absence of ligand. β–arrestin2 recruitment to the plasma membrane (CAAX) was not significantly different for each PTH1R mutant vs. PTH1R-WT (p > 0.05), whereas recruitment to early endosomes (FYVE) was reduced for PTH1R-R485X (Emax as fold-increase from baseline = 1.3 ± 0.1 vs. 6.08 ± 1.2 for PTH1R-WT (P = 0.02, Student’s t test). Data are means ± SEM from three (FYVE) or five (CAAX) independent experiments.
We further evaluated PTH(1-34)-induced recruitment of β-arrestin to the receptors using bioluminescence resonance energy transfer (BRET) biosensors. We thus analyzed the movement of a β-arrestin2 construct tagged with renilla luciferase-II (β-arrestin2rLucII) to either the plasma-membrane or to endosomes, as detected by a renilla green fluorescent protein (rGFP) containing a CAAX or FYVE anchoring motif, respectively43. As shown in Fig. 5c, PTH(1-34) stimulation of PTH1R-WT resulted in a robust recruitment of β-arrestin2rLucII to both the plasma-membrane and to endosomes, whereas stimulation of PTH1R-R485X resulted in markedly blunted responses, especially to endosomes (fold-BRET increases from baseline, WT vs R485X, 4.79 ± 0.85 vs. 2.69 ± 0.65, P = 0.10 at the plasma membrane; 6.08 ± 1.21 vs. 1.33 ± 0.13, P = 0.02 at endosomes). These findings are consistent with the C-tail of the PTH1R playing a key role in stabilizing interaction of the receptor with β-arrestins and enabling translocation to endosomes12,13,14,16. PTH1R-Y134SA exhibited a modest reduction in capacity to recruit β-arrestin2rLucII to the plasma membrane while recruitment to endosomes was comparable to that of PTH1R-WT (Fig. 5c). The responses of PTH1R-E35K for both the plasma membrane and endosomal reporters were similar to those of PTH1R-WT. These BRET data thus concur with our fluorescent microscopy analyses, as they reveal a marked deficiency in β-arrestin2 recruitment to endosomes for PTH1R-R485X, but not for either PTH1R-Y134S or PTH1R-E35K in response to PTH(1-34).
We also noted upon direct inspection of the time course data obtained in these BRET assays that the plasma membrane recruitment response for PTH1R-R485X tended to exhibit a shallower initial phase (t = 0 to 5′) and hence a slower rise to peak signal, as compared to that of PTH1R-WT, while, as expected, no endosomal recruitment signal was detected for PTH1R-R485X at any time point (Supplemental Fig. 6). These data thus seem consistent with the notion that PTH1R-R485X forms only relatively low affinity complexes with β-arrestin2 that assemble relatively slowly at the plasma membrane and then are not stable enough to transit to endosomes, which is also suggested by a recent study on the PTH1R that utilizes cells lacking G protein receptor kinases (GRKs) that mediate receptor phosphorylation25.
We then assessed by fluorescent microscopy in GBR24 cells the capacity of the PTH1R variants to recruit β-arrestin2YFP translocation in response to PTHrP. Treatment of PTH1R-WT with PTHrP(1-36)TMR resulted in robust co-clustering of the ligand in endosomes with β-arrestin2YFP, while treatment of each PTH1R Eiken mutant resulted in generally weaker staining of the ligand in endosomal clusters and there was no co-clustering with β-arrestin2YFP (Fig. 6a, b and Supplemental Fig. 5b). A lack of clustering of β-arrestin2YFP into endosomes with each PTH1R mutant, and a robust clustering with PTH1R-WT was also observed in cells treated with unlabeled PTHrP(1-36) (Supplemental Fig. 7). In contrast, Ile5-PTHrP(1-36)TMR induced a robust co-clustering of β-arrestin2YFP with the ligand in endosomes with each PTH1R variant except PTH1R-R485X, for which β-arrestin2YFP again remained dispersed in the cytoplasm (Fig. 6c, d and Supplemental Fig. 7). BRET analyses performed with PTH1R-E35K revealed that Ile5-PTHrP(1-36) was nearly 10-fold more potent than PTHrP(1-36) and as potent as PTH(1-34) for inducing recruitment of β-arrestin2rLucII to both the plasma membrane and to endosomes (Fig. 6e, f), confirming the profound rescue effect of the His5–>Ile substitution on the capacity of PTHrP(1-36) to promote stable interaction with β-arrestin2 and the recruitment of the complex to endosomes via the PTH1R-E35K mutant receptor.
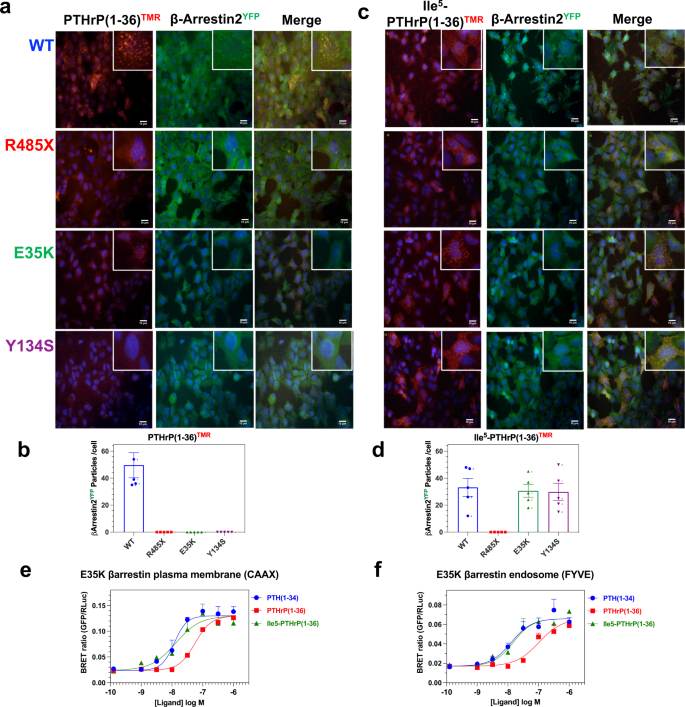
a GBR-24 (HEK293/β-arrestin2YFP stable) cells transiently transfected to express a PTH1R variant were treated with PTHrP(1-36)TMR or Ile5-PTHrP(1-36)TMR (30 nM) for 30 min, then rinsed, fixed and imaged by fluorescence microscopy. The insets show 3X-expanded views of selected cell regions to highlight co-localization of PTHrP(1-36)TMR (red) with β‐arrestin2YFP (green) into distinct clusters (endosomes), with PTH1R-WT but not with PTH1R-E35K, PTH1R-Y134S or PTH1R-R485X. b Quantification of β-arrestin2YFP-positive clusters in five cells positive for binding PTHrP(1-36)TMR for each PTH1R variant. c Cells treated with Ile5-PTHrP(1-36)TMR and imaged as in panel a. d Quantification of β-arrestin2YFP-positive clusters in five cells positive for binding Ile5-PTHrP(1-36)TMR for each PTH1R variant. e and f) BRET analyses of β-arrestin2 recruitment to the plasma membrane (CAAX) and to early endosomes (FYVE) in HEK293 cells expressing PTH1R-E35K and BRET donor and acceptors (as used in Fig. 5c) upon treatment with PTHrP(1-36), PTH(1-34) or Ile5-PTHrP(1-36). Data are representative of three independent experiments (a-d); or means ± SEM of three or four independent experiments (e, f). Bar heights in graphs of panels b and d indicate the means ± SEM of counts from the five cells shown by the individual data points. Scale bars in a and c indicate 10 μm.
These studies overall thus show that the R485X Eiken mutation strongly impairs the capacity of the PTH1R to translocate β-arrestin2 to endosomes in response to either PTH or PTHrP ligands, and that the E35K and Y134S mutations impair the β-arrestin translocation response more selectively to PTHrP than to PTH. Although the mechanisms by which these two mutations in the ECD impact the capacity of the PTH1R to interact with cytoplasmic β-arrestins is not known, studies on other GPCRs have shown that stable interaction with β-arrestin is dependent on a strong interaction with an agonist ligand16,44, which our data suggest does not occur for the PTH1R-Y134S and -E35K mutants and PTHrP.
Impaired PTHrP-induced receptor desensitization for PTH1R variants of Eiken syndrome
β-arrestins are generally thought to play key roles in mediating short-term receptor desensitization and thus limit responsiveness to persistent as well as repeated ligand exposure45. We therefore investigated the capacities of the Eiken PTH1R variants to desensitize cAMP signaling responses induced by PTH(1-34), PTHrP(1-36) as well as LA-PTH during both an initial ligand exposure phase and, after initial ligand washout, during a subsequent ligand rechallenge phase. Transfected GS22a cells were thus pre-stimulated with ligand (1 nM) or with vehicle and cAMP generation was monitored for 14 min (Supplemental Fig. 8a). The cells were then rinsed to remove unbound ligand and cAMP generation was monitored for an additional 90-min washout period (Fig. 7a and Supplemental Fig. 8b). The cells were then treated again with the same test ligand used for initial stimulation or with vehicle, and cAMP generation was monitored for a final 60-min re-challenge period (Fig. 7b and Supplemental Fig. 8b). The level of residual cAMP signaling at the end of the washout period was significantly reduced for PTH(1-34) in cells expressing PTH1R-Y134S, as compared to in cells expressing PTH1R-WT, whereas it was significantly increased for PTHrP(1-36) in cells expressing PTH1R-R485X, and elevated for all PTH1R variants with LA-PTH treatment (Fig. 7a and Supplemental Fig. 8b). The responses observed upon ligand rechallenge, assessed relative to the corresponding responses in vehicle-pre-treated cells, were significantly blunted for each test ligand in cells expressing PTH1R-WT or PTH1R-R485X, while they were blunted only for PTH(1-34) and LA-PTH, but not PTHrP(1-36) in cells expressing PTH1R-E35K or PTH1R-Y134S (Fig. 7b and Supplemental Fig. 8c). These results reveal for each Eiken PTH1R mutant a defect in either signal termination (R485X, Fig. 7a) or signal desensitization after ligand rechallenge (E35K, Y134S, Fig. 7b) that was apparent with PTHrP(1-36) but not PTH(1-34). Of note, these effects mirrored the reductions in the capacities to recruit β-arrestin2 to endosomes, which for PTH1R-Y134S and PTH1R-E35K were specific for PTHrP (Fig. 6a–d). They are also consistent with the notion that PTHrP normally signals only transiently from the plasma membrane and not from endosomes, whereas PTH can signal for more extended times even with internalization to endosomes46. We also note, however, that while a blunting of the ligand rechallenge response is consistent with receptor desensitization, some of the ligand-receptor pairs tested in this experiment maintained at the time of ligand rechallenge a considerable level of residual cAMP signaling that was derived from the initial ligand pre-treatment phase. This was especially noticeable with LA-PTH and likely reflects a residual level of ligand occupancy on the receptor, which hinders any direct mechanistic interpretation of the blunting effects on the ligand rechallenge responses.
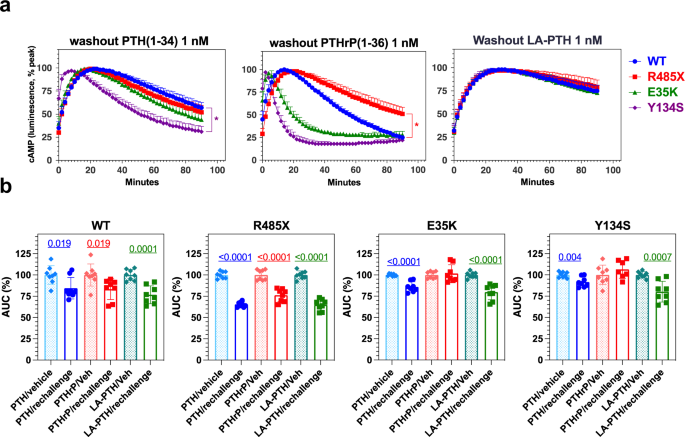
a Assays were performed in Gs22a cells transiently transfected to express PTH1R-WT or a mutant PTH1R. a Duration of initial cAMP signaling response. Cells were pre-treated with PTH(1-34), PTHrP(1-36), or LA-PTH ligand, each at 1 nM concentration for 14 min (pre-treatment responses are shown Supplemental Fig. 8a), and then after washout to remove unbound ligand, cAMP signals were recorded for an additional 90 min. The cAMP signals are presented as the percentage of the maximal signal observed during the washout-period for each peptide on each receptor. The response duration, as assessed at the last 90-min time point and compared to PTH1R-WT, was reduced for PTH(1-34) on PTH1R-Y134S and sustained for PTHrP(1-36) on PTH1R-R485X. P vs. PTH1R-WT response at 90′, * < 0.05. b. Response to Ligand rechallenge. After the washout-periods shown in panel a, the cells were rechallenged with the same ligand (1.0 nM) for 60 min and cAMP accumulation was assessed. Column heights and indicate the means ± SEM of the AUCs of the responses observed for each receptor-ligand pair during the 60 min-re-challenge phase, normalized to the AUC of the response in the respective vehicle-pre-treated cells, points indicate the individual data values. A reduction in the response in the ligand pre-treated and re-challenged cells vs. the response in vehicle-pretreated cells indicates desensitization and is observed with PTH(1-34) and LA-PTH on each receptor, but with PTHrP(1-36) desensitization is observed only on PTH1R-WT and PTH1R-R485X and not on PTHR-E35K or PTH1R-Y134S. P values indicate comparison by Student’s t test to pre-vehicle treatment: Data are means ± SEM from four separate experiments each in duplicate (b) or quadruplicate (a). The corresponding time vs. cAMP-dependent luminescence responses for these studies are shown Supplemental Fig. 8.
Inverse agonist reduces basal cAMP signaling by PTH1R-R485X
Finally, we evaluated whether the elevated basal cAMP signaling activity observed for PTH1R-R485X could be suppressed by treatment with [Leu11,dTrp12,Trp23,Tyr36]-PTHrP(7–36)NH2 {dTrp12-PTHrP(7–36)}, which functions as an inverse agonist on PTH1R-H223R and other constitutively active mutant PTH1Rs of JMC34,41,47. Addition of this ligand to Gs22A cells expressing either PTH1R-H223R or PTH1R-R485X, resulted in rapid decreases in the intracellular cAMP levels for each receptor, as well as dose-dependent inhibition of cAMP signaling induced by a subsequent addition of PTH(1-34) agonist peptide (Fig. 8a, b and Supplemental Fig. 9a, b).
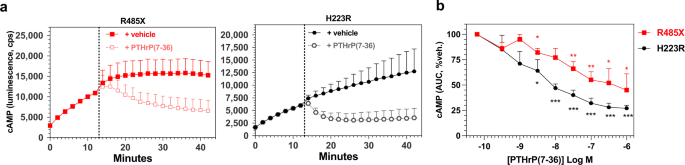
a Gs22a cells transiently transfected with PTH1R-R485X or PTH1R-H223R were monitored for basal cAMP-dependent luminescence for 12 min after luciferin addition (t = 0), and for a subsequent 30 min after addition (at t = 13′, dashed line) of either vehicle or inverse agonist [Leu11,dTrp12,Trp23,Tyr36]-PTHrP(7-36)NH2 (100 nM). b Dose-response relationship of the inverse agonist effect on basal cAMP levels: AUCs of the time vs. cAMP luminescence responses observed between 14 and 26 min in the experiments conducted in panel a were calculated for cells treated with varying concentrations of PTHrP(7-36) analog and normalized for each receptor to the AUC observed in the absence of ligand (−10.5 Log M x-axis data point). Data are means ± SEM of three separate experiments, with triplicate wells in each. Asterisks indicate P values vs. the AUC in absence of ligand for each receptor: *<0.05; **<0.01; ***<0.001 determined by Student’s t test. Additional data from this experiment are shown in Supplemental Fig. 9.
In studies aimed at parsing out whether PTH1R-mediated signaling responses were derived from the cell surface or from endosomes, we assessed the capacity of dTrp12-PTHrP(7-36), as a membrane impermeable antagonist peptide, to inhibit the signaling response induced by previously applied PTH(1-34)31,48. We found that addition of dTrp12-PTHrP(7-36) (1 μM) to cells 15 min after a prior addition of PTH(1-34) (0.5 nM) resulted in little if any change in the cAMP response induced by the agonist in cells expressing either PTH1R-WT or a mutant derivative, even if the cells were pre-treated with the internalization inhibitor Dyngo4A (Supplemental Fig. 9c). In contrast, addition of the presumably membrane-permeable small molecule PTH1R antagonist, SW10634,49, significantly reduced the cAMP response mediated by previously added PTH(1-34) in cells transfected with any of the tested PTH1R variants, except PTH1R-H223R, for which the bulk of the cAMP signal is likely derived from constitutive receptor activation (see above) and SW106 is not an inverse agonist on PTH1R-H223R34 (Supplemental Fig. 9d). That PTH(1-34)-induced cAMP signaling mediated by PTH1R-WT and the Eiken mutant receptors can be inhibited by a subsequent challenge with SW106 but not dTrp12-PTHrP(7-36) is consistent with the notion that this signaling is derived mainly from endosomes31. It also seems possible, however, that the higher affinity, and hence slower off rate with which PTH(1-34) binds to the PTH1R, relative to dTrp12-PTHrP(7-36), prevents effective displacement of the agonist from the receptor by the antagonist. Although the two peptides bind to at least partially overlapping sites in the receptor’s orthosteric pocket, such inefficient inhibition could in part reflect the relatively short time frame of the assays, even for complexes that are fixed at the cell surface, as we sought to achieve with Dyngo4a pre-treatment. SW106, on the other hand, likely binds to an allosteric site in the receptor49, and so could promote a rapid release of bound PTH(1-34) from the complex, independent of its subcellular location.